Series Overview: Hi, my name is Shari and like the majority of our readers I don’t have a degree in science. What I do have is almost a decade of experience working closely with Genetic Counselors, and a passion for sharing genomic information in an approachable and easy to understand way. This passion was developed early in my career, fueled by my quickly building frustration about the over-complicated resources I found during initial attempts at self-guided learning. Genomics 101 is a blog series by GenomicMD that aims to be a solution to that frustration for others. By breaking down the complex language surrounding this field, we hope to empower people (like you!) to be more informed about how genomics can affect their healthcare journey.
Welcome back to Genomics 101, GenomicMD’s ongoing blog series where we help our readers cut through complicated scientific language and build a strong foundational knowledge of genomics with a little bit of fun at the center. In our last post we discussed the history of heredity and introduced you to the ‘father of genetics’ Gregor Mendel, as well as a few of his theories. In that blog we were able to introduce a basic definition of inheritance and also review the concept of dominant and recessive alleles. A full and well-rounded understanding of heredity requires a bit more information than we can fit into a simple definition, so our next few posts will expand on this concept and help our readers appreciate what inheritance is, define some of the more commonly used terms associated with it, describe how this all plays into ancestry and ethnicity, and explain why it’s so important to understand this stuff when discussing genetic risk. Let’s get started!
In our last Genomics 101 post we briefly defined the concept of heredity as how organisms pass genetic information down from parent to child, as well as explained how dominant and recessive alleles came to be understood and defined during Gregor Mendel’s experiments on pea plants. To recap and expand a bit before moving forward, we each receive 2 copies of our genes, one from each parent. An allele is a unit of variation in those pairs of genes and they can be dominant (will consistently produce the same trait even when inherited in a pair with a non-identical allele) or recessive (will not produce a specific trait if inherited with a dominant allele.) While admittedly the genetics of eye color can be complex, we will use it as an example here to explain dominant versus recessive inheritance because it’s familiar to everyone. Brown eyes are determined by a dominant allele, which will overpower both green or blue-eyed alleles. On the other hand, the green-eyed allele is overpowered by brown, but is dominant to blue, while the blue-eyed allele is always recessive. Knowing these traits and their dominant and recessive qualities can help us determine the statistical likelihood of what eye color a couple’s offspring will have. We can visually demonstrate this concept using something called a punnett square, which is a table that can help determine the expected percentage of a given trait being present in the offspring of 2 parents.
The simplest punnett squares are divided into 4 equal sections, with initials for each allele written along the side and top of the table using capital letters for dominant traits, and lowercase letters for recessive traits. See the image below for an example of punnett squares in eye color.
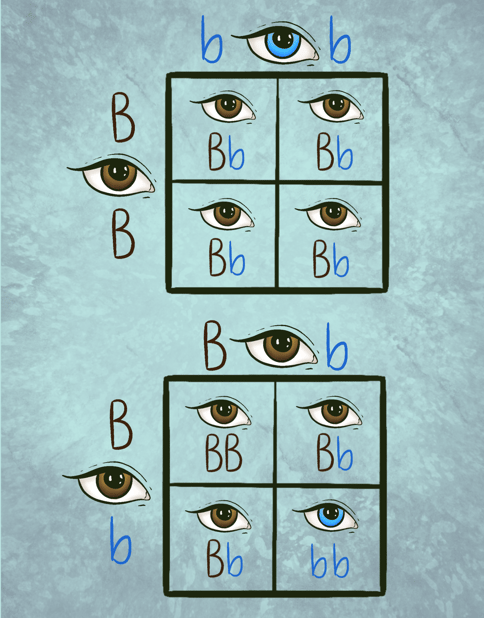
In the first punnett square, you can see that the parent who is listed along the left side of the table has brown eyes, which are noted by listing 2 capital “B”s for each inherited dominant brown-eyed allele. The other parent, who is listed at the top of the table has blue eyes, which are represented by 2 lower case “b”s for each inherited recessive blue-eyed allele. Both of the parents noted in this square are considered “homozygous”, meaning they have inherited two of the same alleles from each of their parents. You can see that when these alleles are added to each section of the square (which represents a possible combination for one offspring) that this match will always create brown eyes. This is noted by the combination always leading to a grouping of one dominant and one recessive allele. The second punnett square represents another type of inheritance involving people who received different alleles from their parents like the offspring in table 1, also called being “heterozygous”. Table 2 shows the statistics for their offspring’s eye color if partners with different alleles decide to have children together. As you can see, both parents have brown eyes which are noted by listing a capital “B” for the dominant brown-eyed allele alongside the lowercase “b,” which represents the recessive blue-eyed allele. When we add these traits to the table individually, we see that there is now a 1 in 4 (or 25%) chance for an offspring to inherit one recessive blue allele from each parent and have blue eyes.
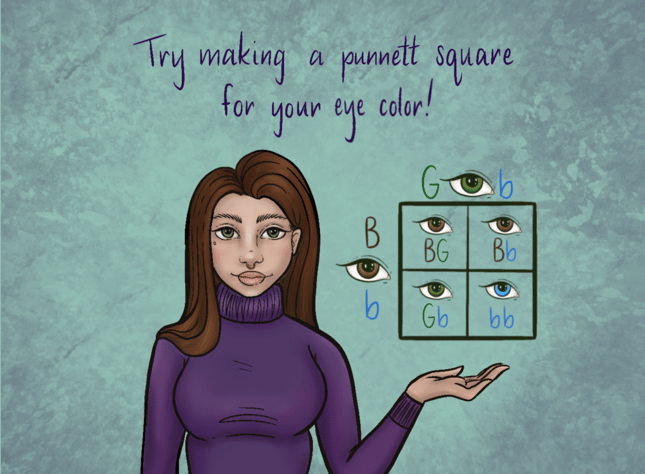
Brown vs. Blue eye color is a great example to explain the basic concept of how some traits are inherited, however certain things about our body (such as risk for disease) involve more complex mechanisms than just whether or not one allele is dominant or recessive. We know this because people with the same genetic variants or mutations in a family will sometimes present with very different phenotypes. This is because not all genetic mutations have the same level of penetrance, or likelihood to create specific symptoms. Some inherited conditions are associated with variants that have 100% (or complete) penetrance. This means that every person who has that specific genetic variant will develop symptoms of the condition in their lifetime. This is true for conditions like Huntington’s disease or neurofibromatosis type 1. Any time a gene’s penetrance is less than 100% it is said to be reduced, meaning that not all people who inherit it will present with symptoms. This is true for certain mutations that present with an increased, but not 100% chance of developing disease, such as certain well known mutations in cancer genes like BRCA1 and BRCA2. The reason for reduced penetration with these mutations is not 100% understood with current science, but it’s believed to be a complex combination of genetic, environmental, and lifestyle factors.
Along with the spectrum of penetrance determining whether symptoms show up, many mutations also have a wide range of expression in terms of how they show up, or whether they show up with mild or severe symptoms. Variable expressivity refers to the range of phenotypes occurring in patients diagnosed with the same genetic condition. For example, a patient with a particular variant related to colon cancer may have milder symptoms (like developing a few polyps that never turn into colon cancer), while another patient with the exact same variant may develop hundreds of polyps that turn into an advanced form of cancer. Variable expressivity is common in both recessive AND dominant conditions, and just as with penetrance we do not 100% understand the reasoning for this phenomenon, but we assume it has something to do with the complex relationship between our genes and our environment. This is one of the foundational reasons why it is so important to know and understand our individual genetic risk factors. If we know our risks and understand our options, we are empowered to take them into account when making lifestyle choices and discussing screening and treatment recommendations with healthcare providers.
All of this goes to say that the mutations you have, though important to the overall picture of your lifetime risk, are not the ONLY factor in whether or not you will develop a certain disease, or even how severe the disease will be if you DO develop it. Variable expressivity and penetrance can alter the severity or expression of a condition, even within the same family. It is important to know your genetic risks so that you can have productive, knowledgeable conversations with your family and healthcare team about environmental risk mitigation, or lifestyle choices that may reduce your likelihood to develop inherited conditions. Thank you for joining us on this journey of learning more about how genes are expressed in our body and determine how diseases develop. Our next chapter will put this information into context with regards to family history and ethnicity, and explain the difference between genetic and genealogic inheritance. We look forward to seeing you there!
Blog Glossary:
Punnett Square - a table that used to help determine the expected percentage or chance of a given trait for the offspring of 2 parents using dominant and recessive alleles
Homozygous - having inherited identical alleles for a given trait from each biological parent. For example with human eye color: someone with blue eyes will always be homozygous for that trait, with 2 identical recessive blue-eyed alleles
Heterozygous - having inherited different alleles for a given trait from each biological parent. For example with human eye color: someone can have brown eyes with homozygous traits by inheriting identical brown-eyed alleles, but they can also have brown eyes and be heterozygous by inheriting one dominant brown-eyed allele and one recessive blue-eyed allele.
Penetrance - refers to the likelihood of a genetic mutation to create symptoms of it’s associated disease. A mutation with 100% (or complete) penetrance will cause symptoms to develop in 100% of people with that mutation.
Variable Expressivity - refers to the range of phenotypes occurring in patients diagnosed with the same genetic condition. It is the reason 2 people with the same mutation may present with different symptoms of severity of disease
Environmental risk mitigation - lifestyle choices that may reduce your likelihood to develop inherited conditions.